Introduction
My goal was to see what a starship would look like if designed and built this century. In prior articles we developed broad outlines of what this ship will look like. Because of the 4000+ year voyage time a relatively large a crewed vessel would be put on a one-way colonizing mission. In order to escape our solar system a high thrust powered maneuver would be performed at the beginning of our journey which limited our vessel mass to about 200,000 mt. Because of the need for cosmic ray protection as well as the need to slow down at our target star, our starship would need to mass 4million mt when cruising.
To achieve this mass along with a 300kps velocity after our powered maneuver, we would begin picking up reaction mass along our starship path and using this material as reaction mass to accelerate. Once we achieved our velocity, we would continue to pick up mass to build out the cosmic ray protection and the mass needed for deceleration at our target.
This article will add some more details to our voyage and the requirements of the packet launching Mega Mass Driver (MMD).
Mass of the Starship Power Supply
We determined that after the powered maneuver our ship will mass approximately 200,000mt. We need to calculate the mass for our power supply. I considered several iterations of a starship attempting to balance mass flow of our mass driver, with the mass of the power supply and finally settled on a one kg mass being accelerated every 5.25seconds (equivalent of .19kg per second). To accelerate this mass, assuming a MD efficiency of 80%, requires a power plant of about 1.2GW. This mass would arrive via a 20kg packet that is intercepted every 105 seconds.
In Part 2 of this series, I stated that the minimal requirement for our power supply would be to generate 20 We/kg of reactor weight. The reason for this now becomes obvious. For a 1.2GW plant our power plant will mass 60,000mt. Add the 38,000mt for our crewed tori, and 25,000mt for our MD, leaves us only 87,000mt for our cosmic ray protection. Even though this is a substantial amount, it would only provide a little more than one meter of water protection- barely 1/6th what would be needed. If our power supply were heavier this would quickly reduce our mass available for cosmic ray protection to zero. Conversely, if our power supply could provide 30 or 40We/kg, we could choose to either accelerate our ship faster or dedicate more mass to our cosmic ray protection.
Using a mass flow of .19kg per second it would take us about 80years to accelerate to 300kps from our starting velocity of 60kps after the powered maneuver. The decision needs to be made as to whether to build out our cosmic ray protection before we accelerate, which would slow down our rate of acceleration so that it would take many centuries, or to put up with the limited protection until our acceleration phase is complete.
Cruise Phase
Once our acceleration phase is complete, we would accumulate mass for building out our starship to address the following:
- Improving on our cosmic ray protection.
- Build a meteoroid shield (called a Whipple Shield) in front of our spacecraft.
- Store reaction material that will be used for the eventual deceleration.
- Replace items consumed or lost.
The assumption is that materials will continue to arrive in at the same rate as experienced during our acceleration- or the equivalent of just under 6000mt per year. To build out our starship to its final cruising mass of 4million mt will require about 634 years.
Certain raw materials of the ship should last for the duration of the voyage with little or no loss. The ship structure should survive the duration. Many other materials will need to be recycled with near 100% efficiency. The biggest items lost will be our atmosphere- over thousands of years some is bound to be lost through hatches and seals, as well as EVAs. However, compared to our 4million mt cruising mass, these replacement quantities are relatively small. Lost Oxygen will be replaced by the electrolysis of water, and lost Nitrogen would be replaced by separating from Ammonia.
The one material that will be consumed with limited recycling abilities is the Uranium needed for power. Depending on our reactor design, fuel swap outs average about one every four years on a civilian reactor or up to every 30 years on Navy nuclear vessels. The primary driver of this frequency is how enriched the fuel is. The useable part of reactor fuel is 235U which with natural uranium is only .7% . Many proposed reactors, including KRUSTY used highly enriched fuel. Enriched Uranium will reduce the amount of reactor fuel mass needed over the life of the voyage, and for planning purposes I assumed that our fuel has been enriched to about 7%. Even with enriched fuel, the amount required is on the order of 20,000mt, or, assuming some reprocessing capability on the Starship, perhaps as little as 10,000mt over the duration of the mission. This would be delivered via our packet supply and then stored until needed.
Our starship will have the following characteristics during the voyage:
Mass mt | Velocity | ||
Stage 1- Mission Start | 582,000 | At start of powered maneuver. Assume Nuclear Thermal Engine with 1000 Isp engine | |
Powered Maneuver | 210,000 | 65 | After 10 radii solar approach |
Stage 2- Acceleration Phase | 200,000 | 300 | After powered maneuver, dispose of sun shield, unneeded engines. Reaction mass starts getting picked up. Assume Mass Driver of 10,000 second Specific Impulse |
Coast | 4,000,000 | 300 | Build up Cosmic Ray Protection and meteoroid shield |
Decelerate | 200,000 | 0 | Target Star Arrival |
Mega Mass Driver (MMD)
Despite the serious engineering challenges presented by our starship, designing and building the MMD is perhaps the most difficult to execute. Besides its large size and mass, even more intimidating is the amount of power it needs to launch packets in rapid succession.
I assumed that the dV of the packets in relation to the starship are about 30mps. I chose this number as it seemed reasonably low enough velocity that could be captured by the starship. However, when our ship is traveling at 300kps, with packets arriving every 105seconds, this means that the packets must be launched by our MMD at 95 per second. With each packet massing 20kg, the driver will be effectively launching 1900kg per second. This requires more electrical power than all the power generated on the earth- at 80% MMD electrical efficiency, we need 107 TW of power.
There are ways of mitigating this incredible power requirement. The simplest is to have the packets approach the ship at 60kps. This reduces the launch rate by half. Total energy required would be the same but the MMD would be functioning for twice as long.
A second way is to reduce our starship velocity. If we decide a peak velocity of 150kps is adequate, we can reduce our launch rate again in half.
The best option is to generate our power over many years and store it until needed. When the MMD is launching at 95 packets per second, it will only need to operate for about 26 days to deliver the 216million packets required. If we were able to save (store) our power generated over 200 months, a power plant only 1/200th this size would be needed. How to store this much power is a large engineering challenge but the solution would likely be storing it in the rotational energy of a large asteroid.
What Would our Starship Look Like?
Some additional characteristics of our starship are the large heat radiators needed for our power. During peak power needed during the acceleration and deceleration phase we need about 1.2GWe from about 3.6GWthermal. This will require large radiator panels since cooling in space is only radiative and not conductive. Depending on the temperature of the cooling fluids and the shapes of the panels will determine the exact size cooling panel size but a quick calculation indicates perhaps 2.2km2 if we assume a average panel temperature of 150C. These would be positioned down the spine of our ship along the mass driver. The mass of these panels would be included in our power plant mass and is part of the 20We/kg.
Even though impact with any object larger than dust will be infrequent, building of a shield in front of the spacecraft would be prudent. A .1kg object traveling at 300,000mps has tremendous energy- 4.5×109 joules.
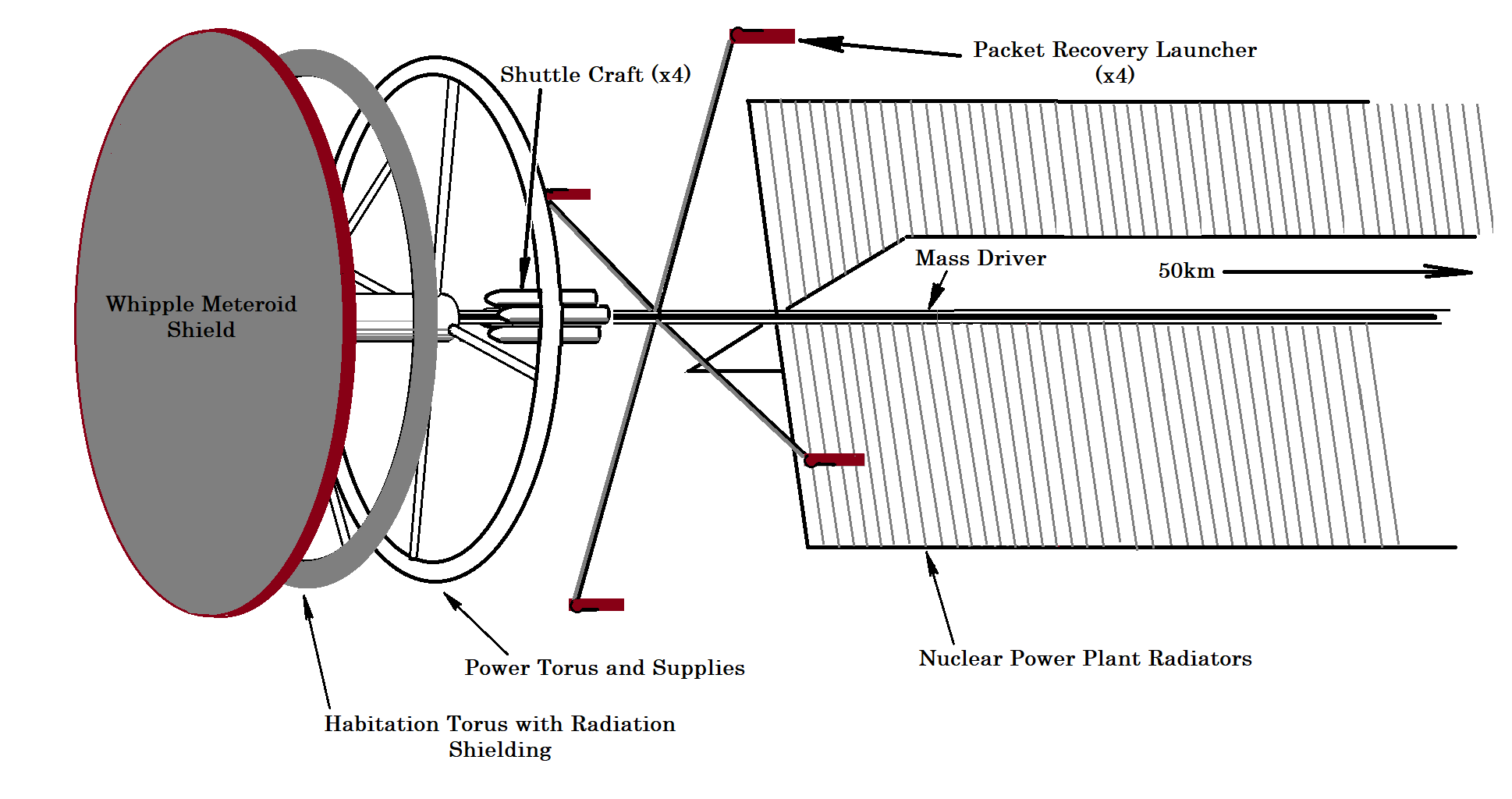
Figure 1 Notional Starship Layout – The Whipple Shied will be 420m in diameter. The habitation torus will have a diameter of 402m. The mass driver will extend the ship almost 50km. The radiator panels will be a cruciform with each panel extending out 200m, and a total radiative area of about 2.25km2
This problem has been looked at before and effective mitigating strategies are available and have been developed. In 1947 F.L. Whipple proposed what has since been named a Whipple shield whereby a thin bumper of metal offset from the spacecraft can protect the underlying spacecraft (Whipple, 1947). When a meteoroid impacts the outer bumper, it and a portion of the bumper vaporizes and dissipates its energy before significantly impacting the underlying spacecraft. With our starship, we will position our mass used for decelerating in front of the ship where it will serve as meteoroid protection until needed for deceleration.
Figure 1 shows a notional view of our starship. Our Whipple shield will have alternate layers of aluminum plating each separated by a couple of meters. Underneath these layers would be a solid base of mixed material that would store our supplies and reaction mass to be used for eventual starship deceleration.
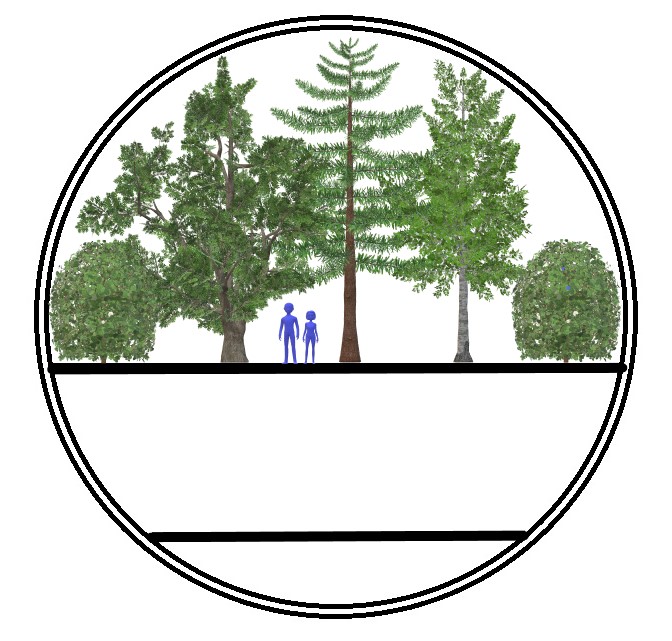
Figure 2 – Starship 15m torus cross section
Our Habitation Torus will have a 402m diameter to the center line to provide a .9g environment. The actual habitation area will have a 15m diameter arranged on two decks. This provides sufficient area to grow crops with additional area for living quarters, office space and recreation. Atmospheric pressure would be 80% earth normal (slightly less than the air pressure in Denver).
Areas for Engineering Improvements
Even though I tried keeping the technology as conservative as possible, there would need to be considerable engineering work to verify this mission architecture is possible. Some of the key technologies required:
- Developing high powered but lightweight fission reactors able to generate at least 20W/kg
- Develop the technology to accelerate buckets and payload at 10,000g with a large MMD
- Develop the packet spacecraft, propulsion and guidance system to ensure accurate target positioning.
- Develop a lightweight MD for our starship that can accelerate a 1kg packet at 10000g. Target mass goal for the MD would be 500kg per meter.
The industrial requirements are even more substantial. The largest are:
- Develop very large space-based manufacturing capabilities
- Design and build a very large 440km long MMD
- Develop vary large power storage system to power the MMD. As conceived in these articles, the starship the MMD may be sending out up to 95 packets per second. If our starship does not use an MD where we assume all the packet mass that is captured by the ship can be used as reaction mass, and instead use electric propulsion with a noble gas, then the number of packets will approximately double.
The two technologies that could dramatically change our mission profile and simplify any starship design are Solar Sails and an advanced Nuclear Engine- either an advanced fission rocket or a fusion powered one. Because of the unknowns associated with their feasibility, I did not include these in my starship design. If extremely lightweight but strong materials could be made then Solar Sails would drastically modify our mission. Launching millions of small solar sails that carry a small payload could eliminate the need for a large MMD and its associated power supply. Similarly, powerful, relatively lightweight fusion power could eliminate the need for our Starship MD. The fusion reactions could be used to generate both power and thrust. Hopefully over the next few decades both technologies will advance enough that a revised starship design can be considered.
Conclusion
It appears technically feasible to build a starship without any fundamental new technologies though it would be extremely difficult. If designed now, the starship would have the following characteristics:
- A two-stage configuration, the first a high thrust engine to perform a powered maneuver to reach a solar system escape velocity, and the second a lower thrust mass driver.
- A 300kps top speed
- The power supply onboard the starship will have to provide at least 20We/kg and it would need to supply about 1.2GWe
- Enroute packet resupply will be needed both to provide reaction mass for our outbound stage 2 acceleration, as well as material to build out our cosmic ray protection, providing some resupply including fuel for our nuclear reactors) and build up the mass for our eventual deceleration.
- An m1 mass of about 200,000 mt for a crew of 1000 and an m0 mass of about 4million mt.
With all these characteristics, our starship would still require a voyage time of over 4300 years to the nearest star.
Despite the possible technical feasibility, the space based industrial requirements are substantial and will not exist for many decades.
Designing a starship is complicated and this article only covers some of the highlights. Further details, including assessments of other technologies, and the many additional challenges that were not addressed here, are discussed on my website and the draft book at https://www.allthingsspace.info.
About the Author: David W McGuire is a retired veteran from the USAF and AF Reserve where he held various jobs as an Acquisition and Procurement Officer, C-5 Maintenance Officer, Maintenance Squadron Commander and Deputy Group Commander. He retired as a Colonel. He also has worked in the chemical, pipeline and petroleum industries where he held varied positions including Terminal Manager, Regional Accountant Manager, Regional Engineering Manager, and Regional Operations Director. He has a degree in Aerospace Engineering and an MBA.